Difference between revisions of "Förster Resonance Energy Transfer"
(→Acronym) |
|||
Line 18: | Line 18: | ||
==Acronym== | ==Acronym== | ||
The most frequently used interpretation of the acronym FRET is “Fluorescence Resonance Energy Transfer,” which is incorrect, because fluorescence is not transferred. Correct readings of FRET are “Fluorescence with Resonance Energy Transfer” (preferred by the present author [1]), or “Fluorescence-detected Resonance Energy Transfer” [2], or “Förster Resonance Energy Transfer “ [3, 4]. The phenomenon itself is Resonance Energy Transfer. RET or Resonance Energy Transfer used to be the most popular acronym [5]. Fluorescence serves to detect RET. Förster is the main force behind FRET. | The most frequently used interpretation of the acronym FRET is “Fluorescence Resonance Energy Transfer,” which is incorrect, because fluorescence is not transferred. Correct readings of FRET are “Fluorescence with Resonance Energy Transfer” (preferred by the present author [1]), or “Fluorescence-detected Resonance Energy Transfer” [2], or “Förster Resonance Energy Transfer “ [3, 4]. The phenomenon itself is Resonance Energy Transfer. RET or Resonance Energy Transfer used to be the most popular acronym [5]. Fluorescence serves to detect RET. Förster is the main force behind FRET. | ||
+ | |||
+ | ==Förster’s Contributions== | ||
+ | |||
+ | Förster’s contributions to FRET are references 10 through 15. His 1946-paper [10] is a short preview of his 1948-paper [11]. In this preview he states the main idea in clear non-mathematical language. The 1948-paper is his most cited paper. There he focuses on FRET between like molecules (homotransfer). This type of transfer can be detected as a decrease in the polarization or anisotropy of the fluorescence with increasing concentration. Reabsorption followed by secondary emission contributes to depolarization, but it can only account for a fraction of the observed depolarization. He introduces the separation R0 (later called the Förster distance or Förster radius; he himself called it the “critical molecular separation”), the separation distance between the donor and the acceptor at which the probability for transfer equals the combined probabilities for all other ways to deactivate the excited donor, and expresses this distance in terms of the overlap integral, the index of refraction of the medium, and the lifetime without transfer. From his data and data in the literature he calculates for fluorescein to fluorescein in water: R0 = 5.0 nanometer, and for chlorophyll A to chlorophyll A in ethyl ether: R0 = 8.0 nanometer. He also defines a critical concentration, c0, as the product of known constants and the inverse cube of R0. He made the simplification (actually, a very good approximation) that only photons emitted by the primary molecule are maximally polarized, and the ones emitted by acceptors are completely unpolarized, so that he is able to derive an equation for the polarization as a function of the concentration both for the low-concentration case and the high-concentration case. In the low-concentration limit, only the interaction between the primary molecule and one other is considered, whereas in the high-concentration limit the excitation energy is thought to diffuse away from the primary molecule. Förster also shows polarization-versus-concentration data for fluorescein in glycerin. The agreement between the data and the low-concentration curve is good up to about one-tenth of the critical concentration. Moreover, the data above the critical concentration are close to the curve derived for the high-concentration case. Finally, he briefly discusses the role of energy transfer in photosynthesis in plants where the chlorophyll concentration is about 0.1 Molar and notes that according to the theory the energy of a photon can migrate over about 10,000 chlorophyll molecules after it is absorbed by the primary molecule. His 1959 review [12] is the 10th Spiers Memorial Lecture and a translation of an earlier paper in German (Th. Förster, Z. Electrochem. 53(1949)93-104). This review is very well written and provides a conceptual understanding of FRET with a minimum of equations. He mentions the classic Bowen papers showing that FRET is different from trivial reabsorption. He compares FRET, reabsorption, donor-acceptor complex formation, and collisional quenching. He mentions three possible situations when his theory is not valid: (1) when thermal relaxation is slow, for example, in gases at low pressures, so that transfer may take place directly from the vibrational level obtained by excitation. In this case the transfer will depend on the excitation wavelength; (2) when dipole-dipole interactions are weak because of forbidden transition in the donor or acceptor; and (3) when the donor-acceptor distances are too small. This last possibility is the Dexter transfer mechanism [16]. |
Revision as of 19:22, 15 December 2017
FRET http://photobiology.info/PDF/History%20of%20FRET.pdf https://link.springer.com/article/10.1140%2Fepjh%2Fe2013-40007-9
by Dr. B. Wieb VanDerMeer, Department of Physics and Astronomy, Western Kentucky University, Bowling Green, KY 42101-1077, USA.
FRET is Fluorescence with Resonance Energy Transfer, or Fluorescence-detected Resonance Energy Transfer, or Förster Resonance Energy Transfer. It is an absorption of electromagnetic radiation by one molecule, the “donor,” followed by resonance interaction with another molecule, the “acceptor.” As a result, energy is transferred from donor to acceptor. The two molecules can be different or identical. If the two molecules are different, the process is called “hetero-transfer” (illustrated in Figure 1). If the two are identical we speak of “homo-transfer” (illustrated in Figure 2) and the energy can go back and forth for a while or go to yet another molecule until emission or a radiation-less transition takes place. FRET is extremely sensitive to the distance between the interacting molecules and can be used to visualize interactions and to measure distances between 1 and 10 nanometers.
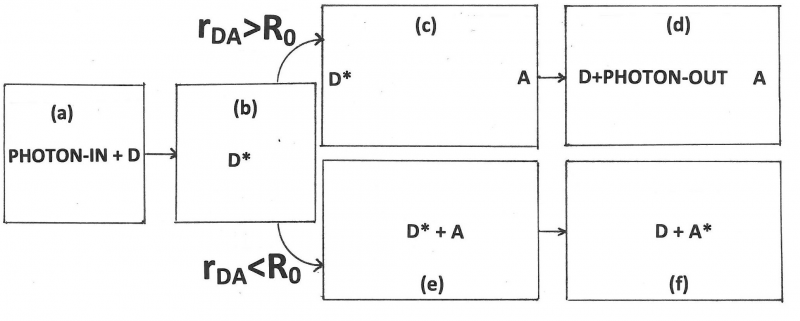

FRET can be observed in different ways. A convenient method in the case of hetero-transfer is to examine the effect of FRET on spectra of donors and acceptors as illustrated in Figure 3.
Contents
1 Acronym
The most frequently used interpretation of the acronym FRET is “Fluorescence Resonance Energy Transfer,” which is incorrect, because fluorescence is not transferred. Correct readings of FRET are “Fluorescence with Resonance Energy Transfer” (preferred by the present author [1]), or “Fluorescence-detected Resonance Energy Transfer” [2], or “Förster Resonance Energy Transfer “ [3, 4]. The phenomenon itself is Resonance Energy Transfer. RET or Resonance Energy Transfer used to be the most popular acronym [5]. Fluorescence serves to detect RET. Förster is the main force behind FRET.
2 Förster’s Contributions
Förster’s contributions to FRET are references 10 through 15. His 1946-paper [10] is a short preview of his 1948-paper [11]. In this preview he states the main idea in clear non-mathematical language. The 1948-paper is his most cited paper. There he focuses on FRET between like molecules (homotransfer). This type of transfer can be detected as a decrease in the polarization or anisotropy of the fluorescence with increasing concentration. Reabsorption followed by secondary emission contributes to depolarization, but it can only account for a fraction of the observed depolarization. He introduces the separation R0 (later called the Förster distance or Förster radius; he himself called it the “critical molecular separation”), the separation distance between the donor and the acceptor at which the probability for transfer equals the combined probabilities for all other ways to deactivate the excited donor, and expresses this distance in terms of the overlap integral, the index of refraction of the medium, and the lifetime without transfer. From his data and data in the literature he calculates for fluorescein to fluorescein in water: R0 = 5.0 nanometer, and for chlorophyll A to chlorophyll A in ethyl ether: R0 = 8.0 nanometer. He also defines a critical concentration, c0, as the product of known constants and the inverse cube of R0. He made the simplification (actually, a very good approximation) that only photons emitted by the primary molecule are maximally polarized, and the ones emitted by acceptors are completely unpolarized, so that he is able to derive an equation for the polarization as a function of the concentration both for the low-concentration case and the high-concentration case. In the low-concentration limit, only the interaction between the primary molecule and one other is considered, whereas in the high-concentration limit the excitation energy is thought to diffuse away from the primary molecule. Förster also shows polarization-versus-concentration data for fluorescein in glycerin. The agreement between the data and the low-concentration curve is good up to about one-tenth of the critical concentration. Moreover, the data above the critical concentration are close to the curve derived for the high-concentration case. Finally, he briefly discusses the role of energy transfer in photosynthesis in plants where the chlorophyll concentration is about 0.1 Molar and notes that according to the theory the energy of a photon can migrate over about 10,000 chlorophyll molecules after it is absorbed by the primary molecule. His 1959 review [12] is the 10th Spiers Memorial Lecture and a translation of an earlier paper in German (Th. Förster, Z. Electrochem. 53(1949)93-104). This review is very well written and provides a conceptual understanding of FRET with a minimum of equations. He mentions the classic Bowen papers showing that FRET is different from trivial reabsorption. He compares FRET, reabsorption, donor-acceptor complex formation, and collisional quenching. He mentions three possible situations when his theory is not valid: (1) when thermal relaxation is slow, for example, in gases at low pressures, so that transfer may take place directly from the vibrational level obtained by excitation. In this case the transfer will depend on the excitation wavelength; (2) when dipole-dipole interactions are weak because of forbidden transition in the donor or acceptor; and (3) when the donor-acceptor distances are too small. This last possibility is the Dexter transfer mechanism [16].